Every Wednesday throughout the academic year, the students and faculty of the Astronomy Department host a public stargazing event from 8-9pm at the Van Vleck Observatory. This event is free and open to the public. Clear skies are mandatory so check the weather first. For more information, click here.
Transit of Venus! June 5th, viewing starts at 5:30pm EDT
The astronomy department will have an open house for the transit of Venus, happening on Tuesday, June 5th. The transit begins just after 6pm EDT and the Sun will set while the transit is still going on. If the weather is clear, we will set up telescopes for safe viewing of the transit. If it’s … Read more
Solar Observing Today, 3/27, until 4pm
Come see the Sun today, March 27, in front of Usdan!
Then join us at 8pm in the Daniel Family Common (3rd floor of Usdan) for the Sturm Lecture by Dr. Alan Title, Director and Senior Fellow of Advanced Technology Center, Lockheed Martin, and Professor of Physics, Stanford University. His talk is entitled “Making the Invisible Sun Visible”. Following his talk, we will observe other celestial objects through the 16″ and 20″ telescopes at Van Vleck Observatory. As with all events hosted by the Astronomy Department, this event is free of charge and open to the public.
Solar Viewing for the Sturm Lecture
In honor of this year’s Sturm Lecture, we will be observing the Sun all day on the lawn outside Usdan center on 3/27 (or 3/26 if the weather doesn’t look great for 3/27). We will observe at visible wavelengths with our 10″ telescope and with our new Coronado H-alpha telescope, as well as our solar spectrograph. Students and faculty from the Astronomy Department will be on hand to answer your questions about the Sun.
Then join us at 8pm on 3/27 in the Daniel Family Common (3rd floor of Usdan) for the Sturm Lecture by Dr. Alan Title, Director and Senior Fellow of Advanced Technology Center, Lockheed Martin, and Professor of Physics, Stanford University. His talk is entitled “Making the Invisible Sun Visible”. Following his talk, we will observe other celestial objects through the 16″ and 20″ telescopes at Van Vleck Observatory. As with all events hosted by the Astronomy Department, this event is free of charge and open to the public.
KH 15D on YouTube
The unique behavior of this “winking star” was discovered in 1995 with the 24 inch (Perkin) telescope at Van Vleck. We continue to follow it at Wesleyan but most data now comes from the SMARTS telescope at Cerro Tololo Interamerican Observatory in Chile. It has been fascinating to see the light curve evolve over the years as precession of the disk has caused various parts of the orbits of the stars to be covered or revealed.
Just recently the star has brightened again, unexpectedly, and it appears that the previously unseen binary component is now peeking out the bottom of the disk. This phase is not shown in the current animation because it was created before we knew this was going to happen.
An interesting aspect of the star is that when it forms a planetary system (IF it forms one!) the planets will probably transit the stars, just like several systems recently discovered by the Kepler satellite, including Kepler 16 (sometimes referred to as Tatooine, after the mythical Star Wars planet orbiting a binary star). KH 15D may, therefore, be described as a proto-Tatooine.
APOW: Jupiter through a historic telescope
Imagine a clear night at the Van Vleck Observatory, you and all your friends have decided to come to a weekly Public Observing Night and take a look at the stars. You walk into the large dome atop Foss Hill and see the magnificent 20″ Alvan Clark Great Refractor before you. It seems to be pointed at a particularly bright star in the sky, and just as you’re about to put your eye to the eyepiece (the above picture shows you almost there), you realize that’s no star, but in fact the planet Jupiter.
You see large bands of clouds, part of the planet’s turbulent atmosphere. Nearby to Jupiter are four other points of light. This time they aren’t stars or planets but instead are Jupiter’s four largest moons. Known as the Galilean Moons or Galilean Satellites, they are named after Galileo Galilei who discovered them in 1610.
From left to right in the image the Galilean Moons are Callisto, Ganymede, Io, Europa. These moons are wonderful specimens in the menagerie of solar system bodies. Callisto is a crater-filled world; Ganymede a moon larger than Mercury; Io a volcanic world locked tight in Jupiter’s gravity; and Europa an ice-covered body with possible liquid oceans underneath. If you’re timing is just right you may even be able to see one or several moons pass in front of Jupiter; from the Jovian cloud tops you’d be privileged enough to see something akin a solar eclipse.
In addition to being an impressive view, Jupiter and its moons have also played an important role in the history of planetary impacts and determining the speed of light.
Speaking of history, the 20″ refractor telescope saw it’s “first light” on 26 July 1922. In the intervening years, up until the 1990s, it was used for research determining the distance to close stars. Distances were determined by a method known as parallax, where you look at the movement of a nearby star compared to much farther ones. A simple example of parallax is looking at your finger through one eye, then the other and seeing its position change compared with objects in the background.
Currently the telescope is used for public observing, as the photographic plates it used for imaging and measurements have mostly gone the way of the dinosaurs (or Pluto’s planet status for that matter). The rosy glow you see around the eyepiece is from lights in the dome tinted red to help preserve your night vision.
Now slaked of your thirst for the celestial, you and your friends leave with a bounce in your step, knowledge in your head and a feeling that you’re king of the world after having seen this most regal of planets.
(N.B.: This image, taken on 1 Dec 2011 ~2109EST, is an overlay of many different exposures, as my camera is not nearly as good as the human eye at adapting to different light levels.)
Summer Research in Astronomy: Wesleyan Students Get Around!
The summer of 2011 was a banner year for Wesleyan students doing research in astronomy. No fewer than SIX of our students obtained competitive REU (Research Experiences for Undergraduates) assistantships sponsored by the National Science Foundation. Alexandra Truebenbach spent the summer in Puerto Rico at the Arecibo Radio Observatory. Phillip Adams and Nora Dumont were in Sunspot, New Mexico at the National Solar Observatory while Alissa Fersch was in Tucson, Arizona at the National Optical Astronomy Observatory. Ben Tweed spent the summer at Colgate University in New York, while David Amrhein split time between Williams College in Massachusetts and Hawaii, where he observed an occultation of Pluto by its moon, Charon.
All of this follows on the heals of a rare experience last year for senior astronomy major Craig Malamut. He joined an expedition to Easter Island to observe a total solar eclipse of the Sun. For more on Craig’s adventures and studies, please see the article about him in the latest issue of the Wesleyan Connection.
If you are a Wesleyan undergraduate interested in astronomy now is the time to start thinking about research for next summer. A good starting place is the Web site of the Keck Northeast Astronomy Consortium. You will find information about KNAC’s REU program and also links to other REU programs across the nation. Also, if you are passing by the poster outside of the classroom in the Observatory, take a look! There are many opportunities available. Application deadlines are approaching, so it is best to start looking now. What better way to spend your summer?!
M is for M-dwarf Models
When Prof. Brian Chaboyer visited from Dartmouth College, we heard all about theoretical models of M dwarfs – the smallest objects that are massive enough to properly be considered stars. Especially, he addressed the issue that for stars of about a half the mass of the Sun, current models predict main sequence stars that are either too luminous or too red by comparison to observations of stars in globular clusters. He explained that observational errors are down to about one percent, but that differences in theories vary by about four percent, and so the choice of model effects the interpretation of the observations. Whereas stellar atmospheric models can be verified by looking at the spectra of stars (Titanium Oxide is typically used to identify M-Dwarfs), models of stellar interiors must map onto the bulk properties of the star such as radius, mass, age and rotation. To measure some of these bulk properties for comparison with his and other models, Prof. Brian Chaboyer studied transiting binary stars and compared his results to computational stellar structure models.
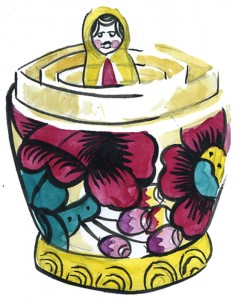
Frenzied globs of gas though they may be, the interiors of stars can be dealt with by taking them to be spheres, composed of many concentric shells, reminiscent of a Matryoshka doll. One wants to know how four particular properties vary from the innermost shell to the outermost, such as pressure, mass, luminosity and temperature gradients. We expect these properties to be interdependent, and they are, which is reflected in four coupled differential equations of stellar structure. Using these equations is akin to asking the following questions:
Question: What change in pressure at each shell would be required to support a star against its own gravity?
Answer:
The Pressure is balanced by the force of gravity, defined in terms of the mass of each shell and the density.
Quenstion: How does the amount of mass differ between each shell?
Answer:
The total mass of the star can be arrived at by summing the mass density at every spherical shell.
Question: How much energy is passing through the surface of each shell at any given time?
Answer:
Luminosity is related to radius, density, and the rate of energy production by nuclear fusion or by changes in gravitational potential energy.
What is the temperature change at each layer?
…. This answer depends on whether energy transport occurs by radiation or convection.
For radiative heat transfer:
Derived by equating expressions for radiation pressure, the change in temperature relates to the opacity at a given shell, the energy flowing through it, and the temperature of the shell. By inspection, we can see that a high opacity and high luminosity give a steep temperature gradient, which is an impetus for convection. The opacities of small, cool stars are challenging because they are cool enough to contain molecules. There is another temperature gradient equation for convective transfer which comes from the first law of thermodynamics, and depends on which equation of state the gas can be considered to have. M-dwarfs are relatively compact stars where effects like degeneracy pressure play a role in the equation of state of the interior. (So said Prof. Pierre Demarque, Prof. Charboyer’s thesis advisor, who was in attendance at this colloquium.)
You can observe for yourself how some of the above properties change for stars of different mass using this applet made available by Dr. Brian Martin of King’s college.
Having a theoretical description of the bulk properties of the stars, Prof. Charboyer used a method employing binary star transits to find their radii. The basic idea was not unlike the now-familiar transit planet-finding method, in that measurements are taken during dips in the light curve when one of the orbiting bodies eclipses the other.
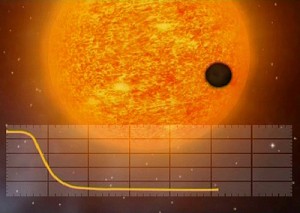
The transit planet-finding method gives the radius of a transiting companion relative to that of its companion. By contrast, Prof. Charboyer used the orbital velocity of the stars and the duration of the eclipse to establish the absolute radius of the objects. Taking the stars to be tidally locked – meaning that their rotational periods are given by their orbital periods – gives a rotation-radius relation that can then be used to fit to models. Prof. Charboyer reasoned that faster rotators would be stronger magnetic dynamos, causing them to have inflated radii, explaining the increased luminosity in observations. He didn’t consider such effects as differential rotation of the stars, but maybe we’ll hear more about that in relation to the Kepler mission targets if we receive a visit from Lucianne Walkowicz later this semester.
M-dwarfs themselves have come to be promising places to find habitable planets, precisely because the ratio of star to planet brightness is lower than for more massive stars. Thanks to the lower temperatures of M-dwarfs, habitable planets could exist close in to the star, their short periods making the chance of catching a transiting Earth-like planet pretty good.